It was Friedrich Nietzsche who wrote: ‘What does not kill me makes me stronger.’ The aphorism offers good guidance during triathlon training and, often, through the tough times in your private life. Does it also apply to the ecosystems of Earth? We think it could. While plant and animal life would be better off without humanity’s climate-altering activities, our planet nonetheless shows resilience and the ability to adapt. That’s hardly an excuse for inaction in the face of the climate crisis, but it is certainly fascinating to predict how the major ecosystems of our home Earth will look in the intermediate and distant future, should atmospheric chemistry and climate change continue along the trajectories seen today. Our main interests are coral reefs, and we want to share some of our thoughts about their future based on our knowledge of unusually stressed ocean ecosystems and evolution. Corals will live on, though in very different form than the ones that thrive now; we also think that the food web will become simplified and dominated by different, much smaller fish.
How will the oceans transition to this new normal? It’s useful to think about coral reefs on a gradient, from the least to the most disturbed. On one end are the pristine, sun-flooded coral reefs well known and loved by scuba divers. This is where impressive towers of hard and soft corals cover almost every square metre of the seafloor, and countless species of colourful reef fish frolic among the intricate three-dimensional structures created by the corals. Because the multiple cracks and crevices between the coral fingers help hide smaller fish from the hungry mouths of predators such as groupers and sharks, the small fish never venture too far from their coral homes. Witnessing such a reef is an enthralling experience for the novice diver and expert marine biologist alike.
These ecosystems thrive in clear water, where nutrient levels are low. Despite these low levels, coral reefs still grow significant amounts of new biomass through two diverse nutrient pathways.
Corals are animals and, in the first pathway, they eat like any animal would. A coral is, simply put, a small sea anemone with a skeleton. Each coral takes the form of an individual polyp and lives in a colony with many other tiny polyps. Each individual coral is a tiny filter feeder that catches plankton (microscopic plants and organisms) for nutrition with its tentacles.
But corals have another means of nutrition as well: they harbour microscopic green algae within their tissue. Algae are aquatic plants, and through the process of photosynthesis, convert sunlight into biological energy, just like any green plant would. In this way, these symbiotic algae power the coral and themselves. Because of the coral’s dual ability to make a living, it’s called a holobiont, a ‘total organism’, incorporating the best of the animal and plant worlds. Especially for many hard corals, which build the most massive calcium carbonate skeletons, the energy derived from photosynthesis makes up the majority of the organism’s energy budget. Hence, clear water, transparent to sunlight, is of paramount importance for the flourishing of a classic coral reef.
Human interference has put all this at risk. In the age of billions of humans, many live in industrialised societies, where multiple environmental stresses are altering tropical marine ecosystems so that they less and less resemble the pristine state of coral flourishing described above.
One activity detrimental to the health of coral reefs is ‘coastal development’, a term that sounds Orwellian to the conservation biologist. ‘Development’ might seem to have a positive connotation, but it actually describes the destruction of coastal ecosystems. Coastal reclamation directly destroys reefs by constructing artificial islands on top of them. And even construction activity on or near the seashore can damage coral reefs: a concrete seawall will filter rainwater flowing into the ocean much less efficiently than an intact mangrove forest. Dense coastal human populations with poor liquid-waste treatment in their communities will make the ocean’s water more turbid and rich in nutrients. These ‘developments’ knock out corals that need clear water for sufficient light to reach their symbiotic algae. In the absence of hard corals, other animals like the soft leather coral Sarcophyton can come to dominate stressed coastlines. Not only does the reduced sunlight harm the hard-coral holobionts; the excessive nutrients serve as fertiliser for other marine algae, often arch-enemies of corals competing with them for space on the reef. Reefs dominated by these algae are a hallmark of many stressed ecosystems in the Caribbean.
The greatest threat to the stability of the planet’s biosphere is human-caused climate change. The massive amounts of carbon dioxide released into the atmosphere as we burn fossil fuels leads to a rise in temperatures. Corals are among the organisms most severely affected by rising ocean temperatures; their physiology is very finely tuned to work at the temperatures of sun-drenched shallow tropical reefs, with an optimum just a bit below 30 degrees Celsius (86 degrees Fahrenheit). Any temperature above that will cause the algae living symbiotically within the coral to produce so much reactive oxygen as a byproduct of their photosynthesis that their coral hosts suffer damage. Responding to that emergency, corals repulse the algae from their bodies. This is the right move in the short term: the corals remain unharmed by the reactive oxygen. But in the long run they will starve without the energy generated by their algal symbionts, and may eventually die if they do not recover symbiont numbers in time. Since the loss of the algae also leads to the loss of the beautiful colours of the corals, this creates the tragedy on the reef known as ‘coral bleaching’. Hard corals are among the most severely affected by this stress.
Reefs can recover from bleaching but, when they do, the survivors are the more resilient colonies and species of corals – or other animals that can survive heatwaves better than the bleached corals altogether. At the same time, many other animals on coral reefs also host photosynthetic symbiotic algae: giant clams, soft corals, sea anemones, zoantharians, sponges, and even some acoel flatworms. Within each of these groups there are species or individuals that are more or less susceptible to bleaching. Understanding what species or individuals are susceptible to bleaching and which are more resilient has been one of the long-standing goals of many coral reef scientists. Although our understanding is far from complete, we can state that susceptibility is a complex and delicate interplay between a host coral’s genetics, those of its symbiotic algae, and local and regional variation in the marine environment.
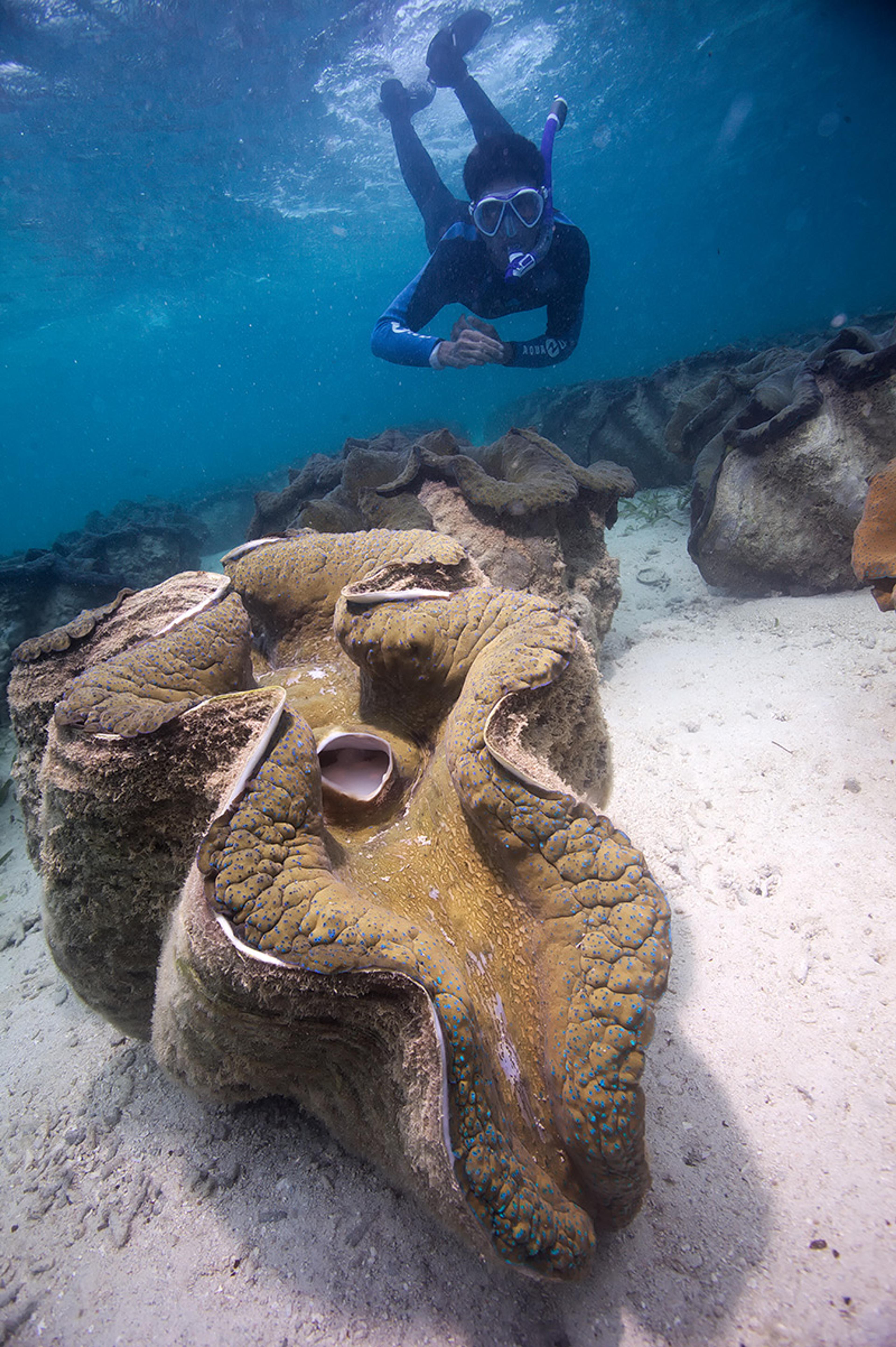
Bleaching events have increased with planetary warming since the 1980s, and we have, unfortunately, learned a lot from the observed coral reef death and devastation. Certain types of corals such as massive Porites are more resilient than the rapid and fast-growing branching genus Acropora. In fact, these two Atlantic species of Acropora are among those listed as threatened under the US Endangered Species Act. We also have learned that the symbiotic algae in corals are not one species as originally thought, but an entire family of hundreds to possibly thousands of different species, each with its own preferred temperature and light conditions. Each coral colony hosts one to a few of these algal species. Often, algal species that provide more energy to host corals can be among the least resilient against warming seawater. In short, depending on the severity of the marine heatwave, the result is a mosaic of bleached and unbleached animals.
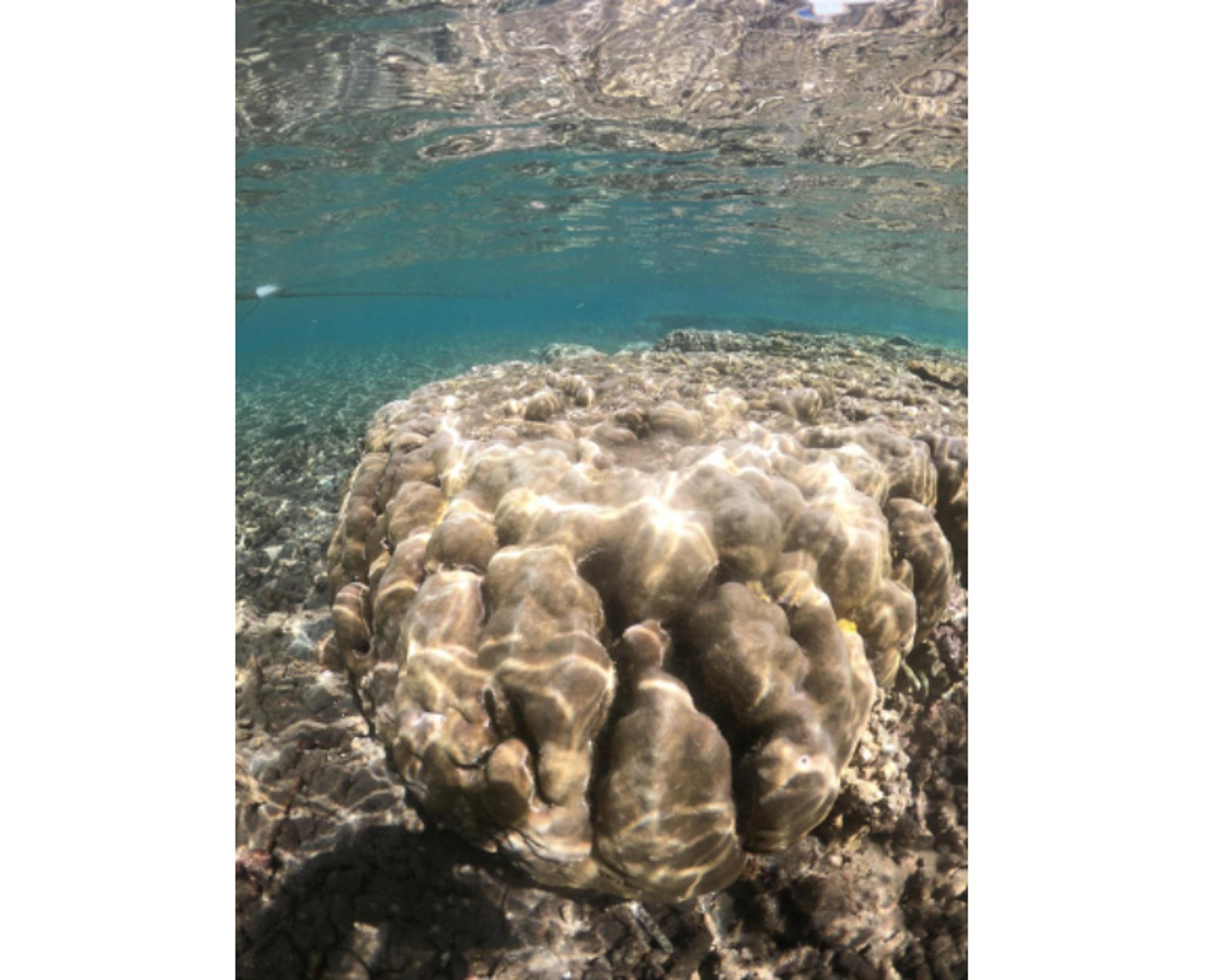
The ‘evil twin’ of climate change is ocean acidification. Here, carbon dioxide originating from the burning of fossil fuels partially dissolves into Earth’s ocean waters; there, it leads to a slight, but significant increase in acidity. Because the precipitation of calcium carbonate depends on precise levels of ocean acidity, it becomes harder for hard corals to lay down their layers of skeleton. At somewhat increased acidity, growth of the coral skeleton slows down and will eventually cease, leading to disintegration of the reefs themselves.
Excessive nutrients introduced by humans favour the growth of algae that outcompete corals for space
One of us (Klaus M Steifel) had the chance to visit some locations in the north of the Philippines where an overload of waste from farmed fishes vastly increased the acidity of seawater. The area was once home to a coral reef but is now devoid of any hard corals. Is this a sad preview of coral life in the future of our home planet, should carbon emissions remain unchecked?
If the Philippines can foretell our future, human activity will ultimately knock out corals with mineralised skeletons or a high reliance on clear water to feed their symbiotic algae with sunlight. On top of that, excessive nutrients in the seawater introduced by humans favour the growth of algae that outcompete corals for space.
Which types of corals will even be left to live in these human-altered seas? There are plenty of coral species that don’t build massive calcium carbonate skeletons, and don’t or only weakly depend on symbiotic algae. Many soft corals come to mind; because of their eight-fold polyp symmetry, they are also called octocorals. These corals often do have skeletons; but unlike the heavy calcium carbonate skeletons of hard coral, theirs are light ones, made of small elements called sclerites and, sometimes, protein.
Other groups of animals living near the sea floor and closely related to stony corals also seem to do well. Among them are naked corals (corallimorpharians), sea anemones, and colonial anemones (zoantharians). In our exploration of the northern Philippines and other severely stressed tropical marine ecosystems, we have encountered high numbers of sea pens – soft corals resembling a feather or a 19th-century ink pen made from a feather, hence the name. Their bottom end is anchored in the ground, and they are specialised for life in sandy and muddy bottoms. They naturally occur in habitats with less than stellar visibility, such as river estuaries and their adjacent reefs. Sea pens have only weak skeletal elements and do not depend on symbiotic algae for their energy budget. The sea pens are hence predestined to thrive in habitats that humans have turned into murky places. Curiously, they provide shelter for a multitude of small shrimp and gobies on a small scale, just like classic coral reefs create a home for many fishes and invertebrates.
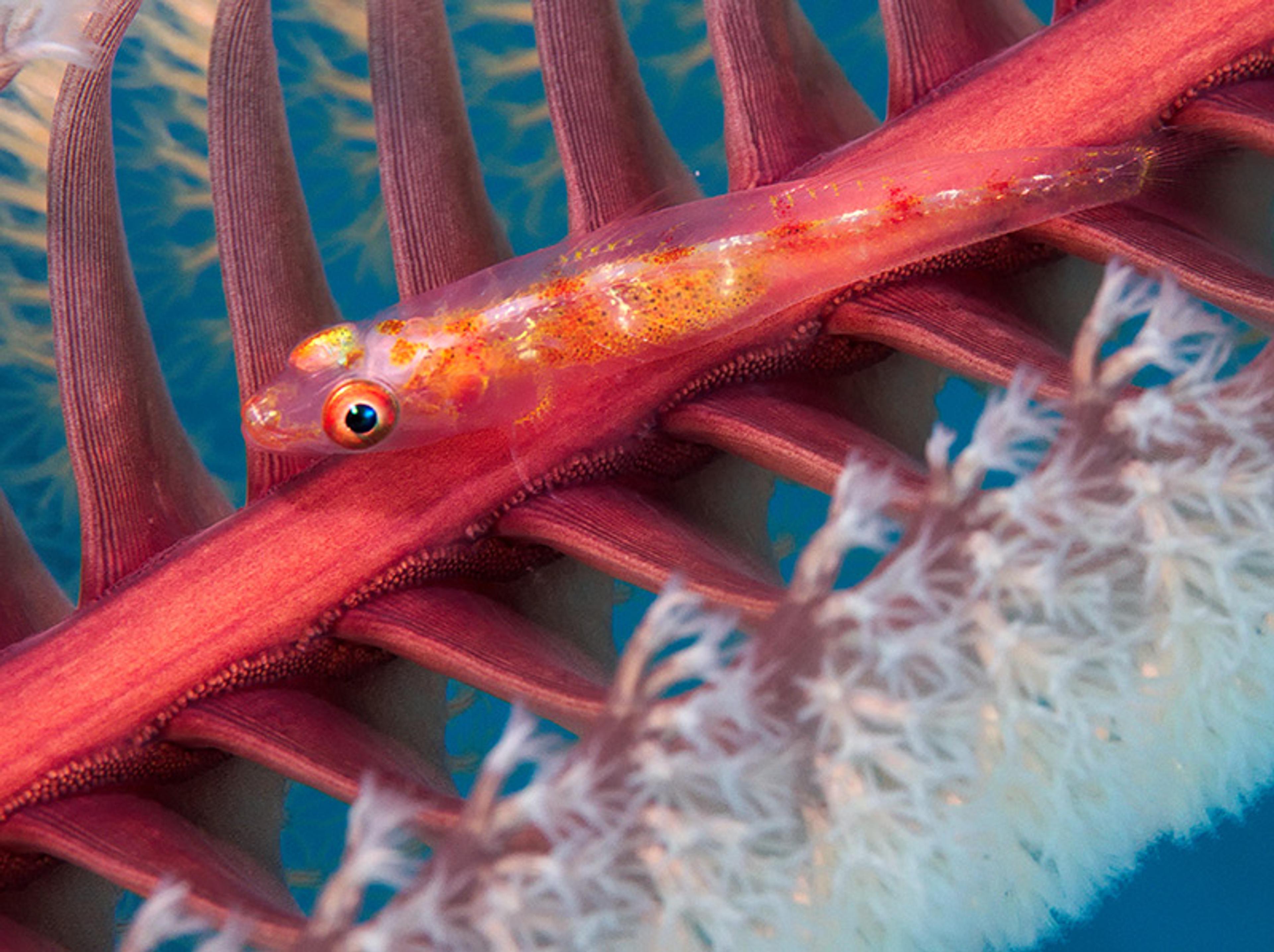
It also appears that some coral relatives, like zoantharians and soft corals, can do well in perturbed environments. In a recent meta-analysis, we looked at approximately 20 locations worldwide – from Brazil to the Caribbean to the East Atlantic to Japan – where local environmental conditions have taken a turn for the worse. We found that many have experienced a shift to high abundances of colonial anemone zoantharians. The colonial anemones in question harbour symbiotic algae like many of their stony coral cousins, but also notably do not produce any calcium carbonate skeleton; instead, they rely on their muscle tissues, and often borrow sand and small particles from the surrounding environment to strengthen their form. Many of these zoantharians are also voracious plankton eaters, which implies that they may be able to survive bleaching events better than species more reliant on symbiotic algae.
Off the coast of Okinawa and Shizuoka in southern Japan, reefs also offer a window into the future. In these unusual ocean ecosystems, where the level of carbon dioxide is unusually high, one finds a unique assemblage of odd, bottom-living species where hard coral ought to be: massive blue Heliopora corals that build skeletons of aragonite (a different crystal structure of calcium carbonate, with more added magnesium than the calcite in hard corals) and their small cryptic cousins Nanipora, as well as selected species of soft corals and zoantharians, along with a smattering of greatly reduced stony coral diversity. All of these animals are found on more normal coral reefs, but why do they do so well at these marginal and comparatively more stressful sites?
The answers remain to be confirmed, but evidence indicates that this eclectic mix of species has the genetic toolkits and abilities to survive and perhaps even thrive in a wider range of conditions than many of their stony coral cousins. This toolkit may include abilities like the potential to be in symbioses with a different suite of symbiotic algae depending on their environment; expanded abilities to withstand short-term extreme hot or cold temperatures; an ability to chow down on plankton; and a myriad of other tricks that make such species more resistant to marginal conditions. In short, such species may not be a master of any one environment but, with a jack-of-all-trades approach, they can survive across a wide range of environments. These so-called ‘generalists’ might be better able to adapt to predicted future coral reef environmental conditions.
The coping mechanisms of corals described so far all work on a fast and local scale. But organisms can adapt much more radically by changing their anatomy and physiology over time – it’s called evolution, and it has happened many times before across hundreds of millions of years.
Before dipping into the evolution of these animals, it’s helpful to delineate the word ‘coral’ itself. While many of us think of the shallow and clear-water coral reefs of the tropics and subtropics, ‘coral’ can have many meanings. These shallow-water corals with symbiotic algae are members of the order Scleractinia, which also includes an equal number of corals without symbiotic algae that can be found on these coral reefs but also into the deep sea and colder marine regions. The order of Scleractinia is part of the class called Anthozoa, which also includes other sibling hexacoral orders: the black corals (Antipatharia), naked corals, sea anemones, and zoantharians, as well as tube anemones (Ceriantharia), and all the various octocorals such as soft corals, fan corals, blue corals, and sea pens. And finally, just to make matters even more complex, there are some corals within an entirely different class, Hydrozoa, including benthic fire corals and lace corals. These animals are part of the same class, and can all be found on shallow coral reefs.
The appearance and diversification of all these different groups, with their booms and busts and their different life strategies, provide us with information on what may be in store for coral reefs of the future. In recent years, scientists have attempted to decipher their messages using genetic analyses to examine when and how these different corals evolved across time, and under what ocean conditions.
We can expect more calcite seas, and thus aragonite-skeleton stony corals could be in trouble
The first major finding from this line of research can be taken as somewhat reassuring. Corals in the broad sense have been around for hundreds of millions of years, evolving somewhere between 900 and 800 million years ago in the Precambrian period, and surviving five major extinction events, unlike many of their marine brethren (hello, trilobites) and terrestrial counterparts (here’s looking at you, T rex). Thus, we can consider it likely that corals in the broad sense will make it through the Anthropocene too, more than we can say with certitude about Homo sapiens. Still, corals didn’t pass through these events unscathed. In each event, the large majority of coral species went extinct, particularly in events known as reef crises, when reef-builders almost completely disappeared due to ocean acidification and/or global warming. However, some species, often deep-sea animals with uniquely adapted skeletal formation strategies or lifestyles, navigated these dramatic times, and subsequently diversified after these periods had passed.
The other important finding from such research is about what types of corals evolved when. Skeletal composition is the key. Across geologic times, oceans have fluctuated between aragonite (high magnesium) and calcite (low magnesium) seas. During aragonite periods, aragonite-skeleton stony corals and blue corals appear to have evolved, as would be expected, while during calcite periods other groups appeared and diversified. Thus, it appears that various groups of corals have had success when the conditions were correct for their skeletons to form easily based on the seawater chemistry.
Looking to a possibly warmer and more acidified future, what does this mean for coral reefs and corals? With increasing ocean warming and acidification, we can expect more calcite seas, and thus aragonite-skeleton stony corals could be in trouble. Other groups, such as octocorals, anemones and zoantharians, may fare comparatively better, and this seems to be occurring already in some reefs.
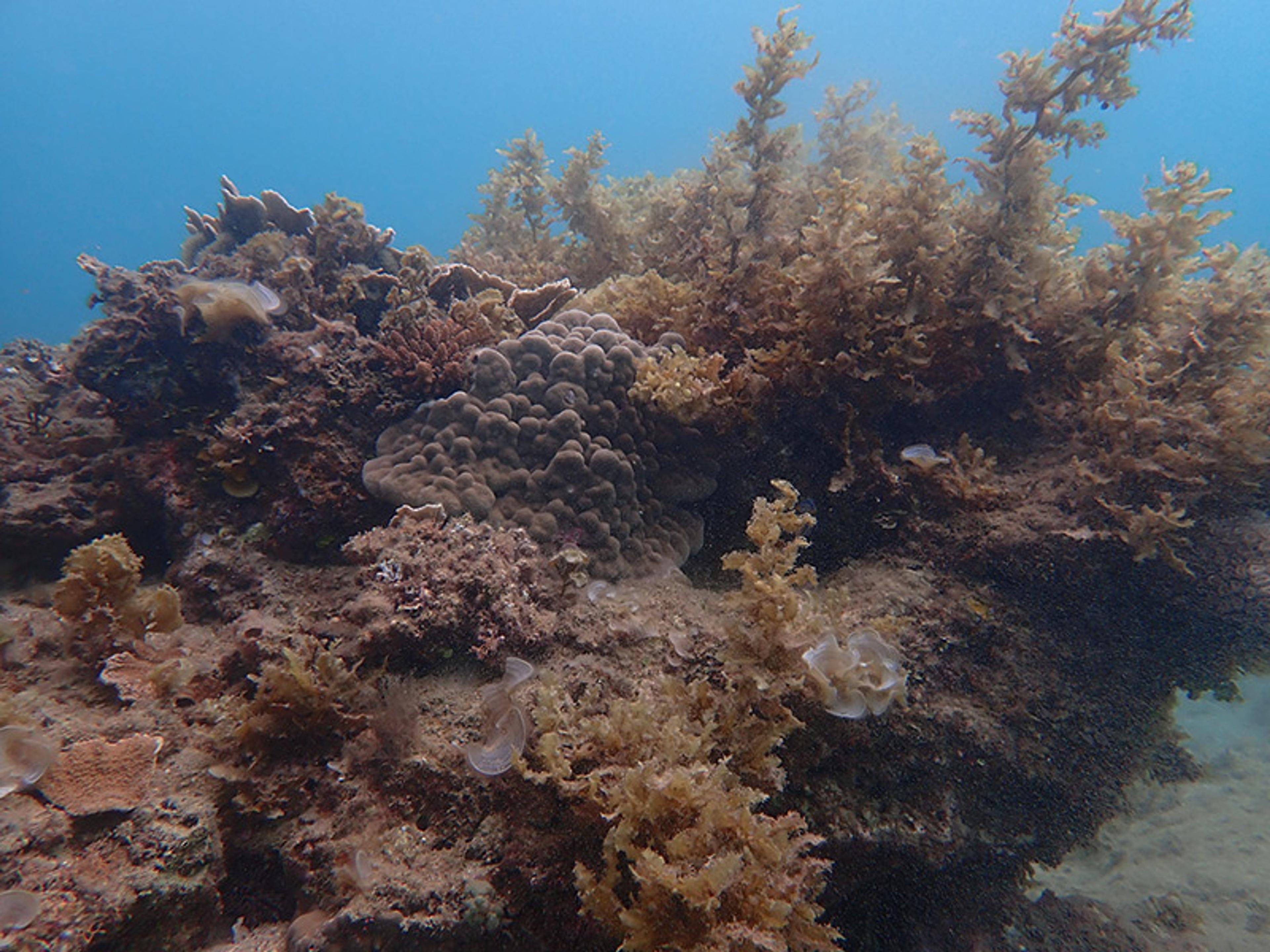
Enigmas remain. For instance, blue corals, with their aragonite skeletons and symbiotic algae, would appear to be losers in future reefs. Yet there are several reports suggesting otherwise. Indeed, our work shows that Nanipora and Heliopora, two species of blue coral, were among the few dominant benthos at high-CO2 sites.
As the hard-rock band Whitesnake sang, could this be a case of ‘Here I go again’ for another round in the evolution of corals? Yes and no. In the long term, the Anthropocene may prove fatal for many stony coral species. However, in the short term, what we are most likely to see is not the extinction of stony corals, but a major shuffling of which corals are common on reefs. Even in areas with comparatively normal carbon dioxide conditions, we may see large shifts to more stress-resistant massive stony corals, along with soft corals, anemones and zoantharians, particularly in areas with declining water quality and rising ocean temperatures. Looking at high-CO2 reefs can give a glimpse into the future, hundreds or thousands of years from now. But, for a quick preview of reefs in the next century or so, a dive in a murky local bay, with decreased water quality and warmer temperatures, might be a better indicator of what our children and grandchildren will see.
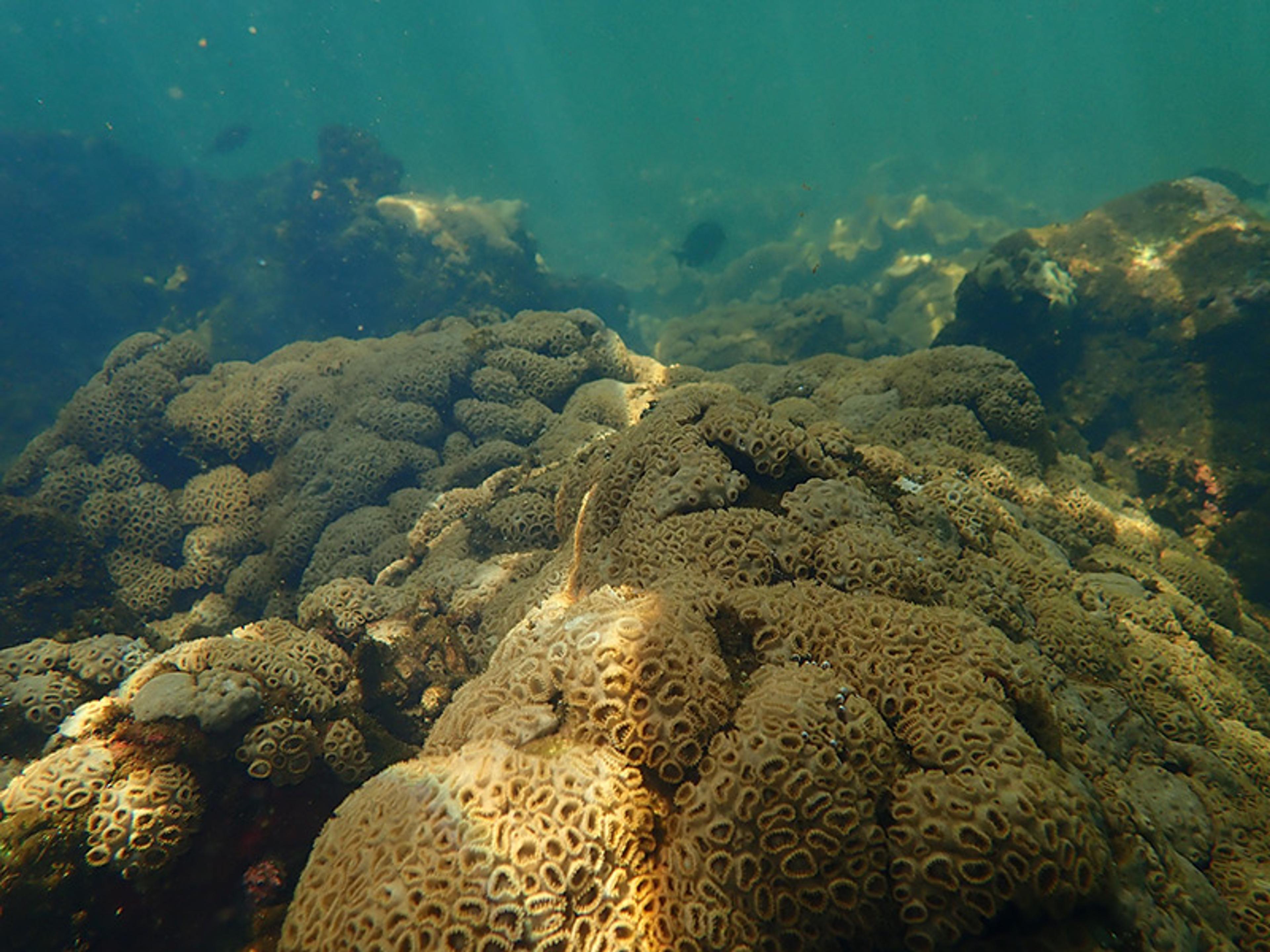
Corals are, in many ways, the cornerstones of marine ecosystems in the tropics and subtropics of our planet. Just like corals, the lives of fishes in marine ecosystems are changing in the face of human domination of Earth’s oceans. Increasingly effective fishing is taking increasingly large numbers of fish out of the oceans in many fishing grounds, and in many cases fish populations have collapsed under this intense pressure – the north Atlantic cod fisheries collapse is an infamous example of the harmful effects of overfishing. In some heavily fished species, humans seem to be driving their life-histories’ evolution: in order to pass on their genes before succumbing to the tightening nets of the fishing boats above them, a number of fish species are now becoming sexually mature at smaller sizes and at younger ages. And just as the Anthropocene is selectively more detrimental to some species of corals than to others, humanity’s fishing pressure is more detrimental to some species: the intensive fishing of long-lived, slowly reproducing fishes like the orange roughy (with lifespans of up to 150 years) can easily wipe out populations of these fishes. Finally, the apex predators of the oceans, the great sharks, are also suffering disproportionally from humanity’s interventions in the ocean ecosystems. These are long-lived species (more than 400 years in the case of the Greenland shark!) that reproduce slowly. Heavy fishing of these majestic animals crushes their populations much more easily compared with, for instance, fast-growing and reproducing sardines, or many of the small cryptobenthic fishes common to coral reefs. Get your great white shark cage-diving in while you can!
We will not leave a sterile planet, but one with significantly less complex and often less beautiful ecosystems
We can make an educated guess that future ocean fish will be diminished in numbers and in species diversity, and will mostly consist of small, fast-growing species, more of them food generalists that can dine on a wide variety of nutrients. We can also forecast that future coral reefs will be more dull-coloured, in line with recent research from the Great Barrier Reef, where the bright colours have already been dulled by bleaching and degradation. In short, a simplified coral reef ecosystem may lead to similar effects in fishes.
Similar changes will likely happen in many other marine ecosystems, too. Moving further away from the tropics and subtropics, we (still) encounter the majestic giant kelp forests. These macroalgae can grow to 45 metres in length, up from the seafloor, and are home to many fishes, crustaceans and marine mammals. But warming ocean temperatures have already decimated the giant kelp in Tasmania, Australia’s southern island state, where the ecosystem is now dominated by a different, shorter-growing kelp species. Giant kelp forests in North America might face similar troubles in the future.
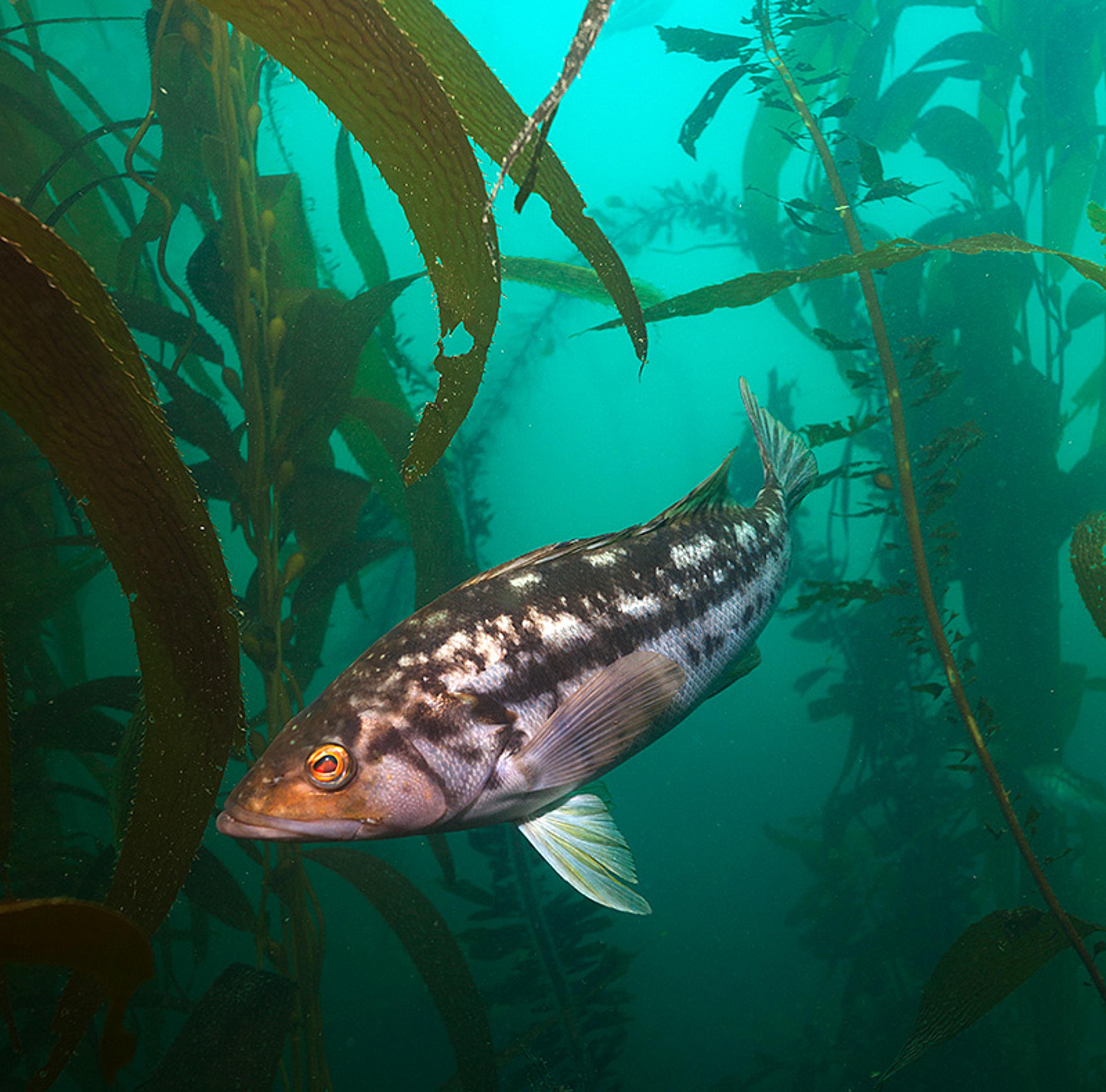
Even further pole-wards, in the Antarctic Ocean, the health of the massive populations of krill, the crustaceans feeding a variety of fishes as well as whales, seals and penguins, is endangered by ocean acidification. In a study in Nature in 2013, the researcher So Kawaguchi and colleagues from the Australian Antarctic Division wrote that ‘unless CO2 emissions are mitigated, the Southern Ocean krill population could collapse by 2300 with dire consequences for the entire ecosystem.’
The likely trend is that many marine ecosystems will be less productive, less biodiverse and more simplified in their food webs and other interactions between animals, algae and plants. Giants like the big sharks or the giant kelp will disappear or become much rarer. We will not leave a sterile planet to future generations, but one with significantly less complex and often less beautiful ecosystems.
It’s a truism that life on this planet will go on, with or without humans, but it’s worth taking a closer look at the specifics of these possible futures. Some 12,000 years ago, our species had just collectively learned to feed itself via agriculture. We are now numerous as never before, and capable of drastically modifying the surface of our planet, and even its atmosphere. How we use these powers will decide if there will be thriving human civilisations in existence in a further 10,000 years. Marine ecosystems and corals will live on, but how they live depends on us: will we bequeath to Earth magnificent coral reefs with thriving fish populations, or murky, acidic and highly altered marine ecosystems with reduced food webs? With a ‘business as usual’ attitude, the climate crisis will turn the oceans into much more drab, less vibrant places – like the bay in the Philippines where acidic oceans caused by fish farming have wiped out all but a few sturdy soft corals.